Photosynthetic processes can be split into two groups, those that are involved in converting light to charge and those that use the chemical/electric potential generated by the former to produce sugar.
The reaction centre (RC) is at the core of the light-to-charge conversion of photosynthesis, both in plants and in bacteria. It is a complicated pigment-protein complex, with many different chemical structures. The proteins (curls) form a scaffold for the cofactors (colourful, hexagonal structures), which are involved in the light-to-charge conversion. Light is captured by pigments, which creates an excitation in a molecule. This excitation is transferred to the reaction centre, rendering the molecule it originates from positively charged. In the RC two coupled molecules called the “special pair” help separate this charge from its original molecule for good.

To better understand this function, some more abstract models have been created, that describe the reaction centre as a Quantum Heat Engine (QHE). A heat engine converts thermal energy to mechanical energy by bringing its working substance (e.g. a gas) from a high temperature state to one with lowered temperature. An example being the Otto cycle that describes a car engine.
Like a normal heat engine, a QHE converts thermal energy (e.g. radiation from the sun) into useful work (a chemical or electric potential). However, instead of going through a continuous spectrum of energies (from high to low temperature), a QHE consists of a cycle through different, discrete energy levels.

Let’s have a look at the model of the special pair proposed by Creatore et. al.
The special pair consists of two donor molecules ( and
) with degenerate energy levels
and
and one acceptor molecule A. The donors interact with light through their dipole moments
which are parallel to each other and of the same magnitude. Their dipole-dipole interaction couples the two molecules together, forming two new excited states
and
. Their dipole moments get added to each other in the same way, resulting in a bright (
) and dark (
) state. Only the bright state can absorb photons to excite energies from the ground state
. To avoid losing this gain in energy to recombination, the excited electrons have to be funnelled off to the acceptor molecule. However, using the coupled energy states as a basis, the transfer of electrons only occurs from
to the acceptor excited state
. The transfer from the higher state is suppressed.
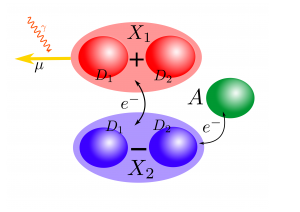
Fortunately, there is an efficient mechanism to move population from the bright to the dark state. By emitting energy into the protein scaffold through vibrations, the excitation can relax down to the dark state and from there move to the acceptor molecule. Now the charge is properly separated from its origin and can be used for work.
Even though the movement through all these energy levels involves a lot more details if looking at the specifics, using this model, the various parts of the process can be studied individually to pin point effects which might increase efficiency.